Faculty

Abdur-Rahman, Jamil
Assistant Professor
Clinical Sciences, Obstetrics and Gynecology
Chicago Medical School
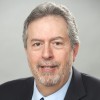
Abel, Marc
Professor Emeritus
Pharmaceutical Sciences, Foundational Sciences and Humanities, Cell Biology and Anatomy
College of Pharmacy, Chicago Medical School
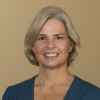
Affinati, Brenda
Director of Clinical Integration, Associate Professor of Medicine
Clinical Sciences, Medicine
Chicago Medical School
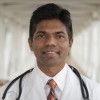
Ahmed, Sohel
Education Director and Assistant Professor
Clinical Sciences, Neurology
Chicago Medical School
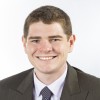
Aitchison, Robert
Clinical Simulation Instructor, Assistant Professor
Physician Assistant
College of Health Professions
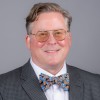
Allswede, Michael
Associate Professor of Emergency Medicine, Assistant Dean of Clinical Education
Emergency Medicine, Clinical Sciences
Chicago Medical School

Anderson, Lori
Chair, Program Director, Department of Nurse Anesthesia
Nurse Anesthesia
College of Nursing

Angelo, Lauren
Associate Dean for Academic Affairs, Associate Professor
Pharmacy Practice
College of Pharmacy

Ariano, Marjorie
Professor Emeritus
Neuroscience
Chicago Medical School, School of Graduate and Postdoctoral Studies
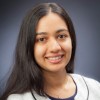
Arshad, Najla
Assistant Professor
Foundational Sciences and Humanities, Microbiology and Immunology
Chicago Medical School, School of Graduate and Postdoctoral Studies
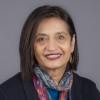
Atre-Vaidya, Nutan
Professor, Vice Dean for Faculty Affairs and Engagement
Clinical Sciences, Psychiatry & Behavioral Science, Neurology
Chicago Medical School

Bair, John
Clinical Associate Professor
Clinical Sciences, Psychiatry & Behavioral Science
College of Health Professions, Chicago Medical School

Baker-Rush, Meredith
Program Director for the IPHS Doctoral program, Interprofessional Research Faculty Lead, DeWitt C. Baldwin Institute
Interprofessional Healthcare Studies, Clinical Sciences, Medicine
College of Health Professions, Chicago Medical School

Bareither, Daniel
Professor Emeritus
Basic Biomedical Sciences
Dr. William M. Scholl College of Podiatric Medicine, College of Health Professions

Barsano, Charles
Professor Emeritus, Graduate Medical Education Advisor
Clinical Sciences, Medicine, Foundational Sciences and Humanities, Cellular and Molecular Pharmacology
Chicago Medical School
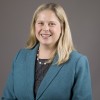
Beaumont, Andrea
Instructor
Pre-Professional Health Sciences, Biomedical Sciences
College of Health Professions

Belmonte, Christina
Assistant Professor
Clinical Sciences, Psychiatry & Behavioral Science
Chicago Medical School

Bernstein, Melissa
Chair, Program Director, Associate Professor
Nutrition
College of Health Professions, Chicago Medical School
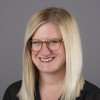
Betten, Elizabeth
Assistant Professor, Director of Clinical Education
Pathologists' Assistant
College of Health Professions

Bilyk, Hope
Assistant Professor
Clinical Sciences, Nutrition
College of Health Professions, Chicago Medical School
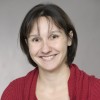
Bottero, Virginie
Adjunct Assistant Professor and Research Associate
Foundational Sciences and Humanities, Humanities & Health Care
Chicago Medical School
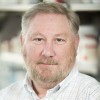
Bradbury, Neil
Professor
Foundational Sciences and Humanities, Physiology and Biophysics
Chicago Medical School, School of Graduate and Postdoctoral Studies
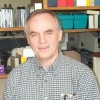
Brandon, Christopher
Professor Emeritus
Cell Biology and Anatomy
Chicago Medical School, School of Graduate and Postdoctoral Studies
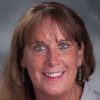
Brennan, Maura
Assistant Education Director & Assistant Professor
Clinical Sciences, Obstetrics and Gynecology
Chicago Medical School

Bridges, Diane
Associate Professor, Assistant Dean for Distance Learning and Curricular Resources
Health Services Administration, Foundational Sciences and Humanities, Humanities & Health Care
Chicago Medical School, College of Health Professions
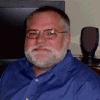
Bridges, Robert
Professor Emeritus and Director of Cystic Fibrosis Research
Foundational Sciences and Humanities, Physiology and Biophysics, Clinical Sciences, Medicine
Chicago Medical School, School of Graduate and Postdoctoral Studies

Broutman, Laurie
Course Director for HBMS502; Medical Director, Pre-Professional Health Sciences Department; Clinical Associate Professor; Chicago Medical School Preceptor
Medicine, Foundational Sciences and Humanities, Biomedical Sciences
Chicago Medical School, College of Health Professions

Buolamwini, John
Chair of Pharmaceutical Sciences, Professor
Foundational Sciences and Humanities, Biochemistry and Molecular Biology, Cellular and Molecular Pharmacology, Pharmaceutical Sciences
College of Pharmacy, School of Graduate and Postdoctoral Studies, Chicago Medical School

Burgess, Kristine
Assistant Professor, Director of Didactic Education
Physician Assistant
College of Health Professions
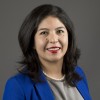
Calderon-Gierszal, Esther
Assistant Professor
Foundational Sciences and Humanities, Physiology and Biophysics
Chicago Medical School
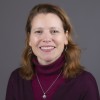
Calkins, Susanna
Director, Nexus for Faculty Success, Associate Professor
School of Graduate and Postdoctoral Studies

Candelario, Danielle
Associate Director of Pharmacy Skills Education, Associate Professor
Pharmacy Practice
College of Pharmacy

Cannady, Preston
Professor
Clinical Sciences, Medicine, Foundational Sciences and Humanities, Microbiology and Immunology
Chicago Medical School

Carlson, James
Interim Provost, Associate Provost for Innovation, Vice President for Interprofessional Education and Simulation, Professor
Interprofessional Healthcare Studies, Physician Assistant
College of Health Professions
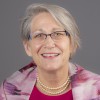
Carvan, Moreen
VP for Academic and Faculty Affairs, Associate Professor
Interprofessional Healthcare Studies
College of Health Professions
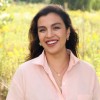
Castañeda, Yvette
Research Assistant Professor, Community Engagement Core Lead, Michael Reese Foundation Center for Health Equity Research
Foundational Sciences and Humanities
Chicago Medical School
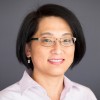
Chai, Weihang (Valerie)
Professor
Microbiology and Immunology
Chicago Medical School, School of Graduate and Postdoctoral Studies

Chang, Kwang Poo
Professor
Foundational Sciences and Humanities, Microbiology and Immunology
Chicago Medical School, School of Graduate and Postdoctoral Studies
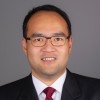
Chatchavalvanich, Santipongse
Assistant Professor
Basic Biomedical Sciences
Dr. William M. Scholl College of Podiatric Medicine
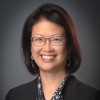
Chen, Melissa
Associate Professor, ICC Clinical Director, Health Equity Content Director, P2H2 I-IV, Assistant Dean for Community Health and Engagement
Clinical Sciences, Medicine
Chicago Medical School
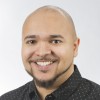
Conkle, Quentin
Assistant Professor
Interprofessional Healthcare Studies
College of Health Professions
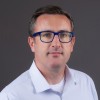
Connaboy, Christopher
Director of CLEAR, Associate Professor
Podiatric Medicine & Surgery
Dr. William M. Scholl College of Podiatric Medicine
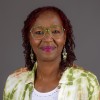
Cooks, Sonya
Chair, Director for the MSN-ENP Program, Associate Professor
Entry into Nursing Practice
College of Nursing

Correll, Carl
Associate Professor
Foundational Sciences and Humanities, Biochemistry and Molecular Biology
Chicago Medical School, School of Graduate and Postdoctoral Studies
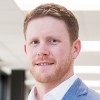
Cotter, William
Assistant Professor; Internal Medicine Residency Associate Program Director (FHCC)
Clinical Sciences, Medicine
Chicago Medical School

Cottreau, Jessica
Chair of Pharmacy Practice, Associate Professor
Pharmacy Practice
College of Pharmacy
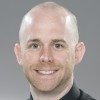
Crews, Ryan
Associate Professor
Podiatric Medicine & Surgery
Dr. William M. Scholl College of Podiatric Medicine
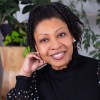
Cross, Kenyotta
Associate Professor & Clinical Counseling Master’s of Science Program Director
Psychology
College of Health Professions
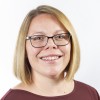
Cunningham, Kathleen
Associate Professor, Director of Clinical Education
Pharmacy Practice
College of Pharmacy

Czukerberg, David
Assistant Professor
Clinical Sciences, Obstetrics and Gynecology
Chicago Medical School
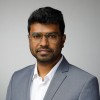
D J, Vidyadhara
Assistant Professor
Neuroscience, Foundational Sciences and Humanities
Chicago Medical School
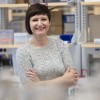
Dabrowska, Joanna
Associate Professor
Foundational Sciences and Humanities, Cellular and Molecular Pharmacology, Neuroscience
Chicago Medical School, School of Graduate and Postdoctoral Studies
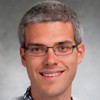
Dahlem, Andrew
Assistant Professor
Clinical Sciences, Family and Preventive Medicine
Chicago Medical School
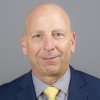
Damaschke, Jeffrey
Chair, Lifestyle Medicine, Director, ICC Clinical & Community Care, Associate Professor
Physical Therapy
College of Health Professions

Dambaeva, Svetlana
Research Associate Professor, Associate Director of Clinical Immunology Laboratory
Foundational Sciences and Humanities, Microbiology and Immunology
Chicago Medical School
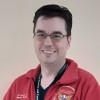
Daniels, Jonathan
Clinical Associate Professor, Learning Community Mentor, Internal Medicine Residency Program Director (FHCC)
Clinical Sciences, Medicine
Chicago Medical School
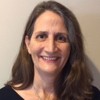
Deamant, Catherine
Professor; Palliative Care Education Faculty Lead
Clinical Sciences, Medicine
Chicago Medical School

Deshmukh, Rahul
Assistant Professor
Pharmaceutical Sciences
College of Pharmacy, School of Graduate and Postdoctoral Studies
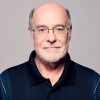
Dickinson, Barry
Assistant Professor
Basic Biomedical Sciences
Dr. William M. Scholl College of Podiatric Medicine

Dillig, Jamie
Director of Outreach and Alumni Relations, Assistant Professor
Office of the Dean
College of Pharmacy

DiMario, Joseph
Interim Vice President for Research, Professor and Dean, School of Graduate and Postdoctoral Studies
Biomedical Research
School of Graduate and Postdoctoral Studies

Dimitrov, Eugene
Associate Professor
Foundational Sciences and Humanities, Physiology and Biophysics
Chicago Medical School, School of Graduate and Postdoctoral Studies
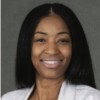
Docheé, Jennifer
Clinical Assistant Professor & Learning Community Mentor
Medicine, Clinical Sciences
Chicago Medical School
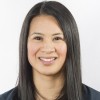
Dookie, Annabelle
Assistant Chair, Associate Professor
Podiatric Medicine & Surgery
Dr. William M. Scholl College of Podiatric Medicine
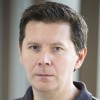
Dundr, Mirek
Associate Professor
Foundational Sciences and Humanities, Cell Biology and Anatomy
Chicago Medical School, School of Graduate and Postdoctoral Studies
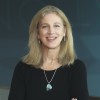
Eliot, Lise
Professor of Neuroscience
Foundational Sciences and Humanities, Neuroscience
Chicago Medical School, School of Graduate and Postdoctoral Studies
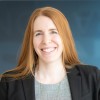
Ellison, Rachael
Assistant Professor
Psychology
College of Health Professions

Evans, Daniel
Professor
Podiatric Medicine & Surgery
Dr. William M. Scholl College of Podiatric Medicine

Everly, David
Associate Professor; Executive Chair of Foundational Sciences and Humanities
Foundational Sciences and Humanities, Microbiology and Immunology
Chicago Medical School, School of Graduate and Postdoctoral Studies
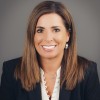
Fasula, Kimberly
Director of Assessment and Interprofessional Education, Assistant Professor
Medical Education, Foundational Sciences and Humanities
Chicago Medical School
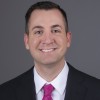
Feinstein, Brian
Professor
Psychology, Foundational Sciences and Humanities, Humanities & Health Care
College of Health Professions, Chicago Medical School

Fennewald, Michael
Associate Professor
Foundational Sciences and Humanities, Microbiology and Immunology, Basic Biomedical Sciences
Chicago Medical School, School of Graduate and Postdoctoral Studies
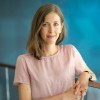
Ferrara, Nicole
Assistant Professor
Physiology and Biophysics, Foundational Sciences and Humanities
Chicago Medical School

Feuerstein, Catherine
Lecturer
Podiatric Medicine & Surgery, Essentials of Clinical Reasoning, Clinical Sciences, Surgery
Chicago Medical School
Fils, Meredith
Assistant Professor & Director of Didactic Education
Physician Assistant
College of Health Professions
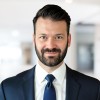
Flanagan, Shawn
Director of Human Performance Lab, Associate Professor
Podiatric Medicine & Surgery
Dr. William M. Scholl College of Podiatric Medicine, School of Graduate and Postdoctoral Studies
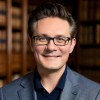
Fleischer, Adam
Professor
Podiatric Medicine & Surgery
Dr. William M. Scholl College of Podiatric Medicine
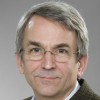
Frost, William
Director, Stanson Toshok Center for Brain Function and Repair; Professor & Discipline Chair, Cell Biology and Anatomy
Foundational Sciences and Humanities, Cell Biology and Anatomy
Chicago Medical School, School of Graduate and Postdoctoral Studies
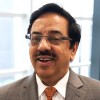
Fulambarker, Ashok
Professor & Pulmonary Medicine Fellowship Director
Clinical Sciences, Medicine
Chicago Medical School

Garber, Sarah
Director of Interprofessional Studies, Professor
Pharmaceutical Sciences, Basic Biomedical Sciences, Interprofessional Healthcare Studies, Foundational Sciences and Humanities, Physiology and Biophysics
College of Health Professions, College of Pharmacy, School of Graduate and Postdoctoral Studies, Chicago Medical School

Gazmuri, Raúl
Professor; Director of the Resuscitation Institute
Clinical Sciences, Medicine, Foundational Sciences and Humanities, Physiology and Biophysics
Chicago Medical School

Gierman-Riblon, Catherine
Associate Dean for Online Learning, Associate Professor and Chair of the Interprofessional Healthcare Studies
Interprofessional Healthcare Studies
College of Health Professions

Gilden, Janice
Professor & Endocrinology Fellowship Director
Clinical Sciences, Medicine
Chicago Medical School

Gilman-Sachs, Alice
Associate Professor Emeritus
Foundational Sciences and Humanities, Microbiology and Immunology
Chicago Medical School, School of Graduate and Postdoctoral Studies
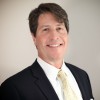
Glaser, Mitchell
Assistant Education Director and Clinical Assistant Professor
Psychiatry & Behavioral Science
Chicago Medical School

Glucksman, Marc
Director, Center for Proteomics and Molecular Therapeutics; Professor & Discipline Chair, Biochemistry & Molecular Biology; Director, Midwest Proteome Center
Foundational Sciences and Humanities, Biochemistry and Molecular Biology
Chicago Medical School, School of Graduate and Postdoctoral Studies
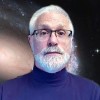
Goldberg, Bruce
Assistant Professor & Learning Community Mentor
Clinical Sciences, Medicine
Chicago Medical School
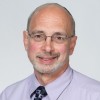
Goldman, Stuart
Professor
Clinical Sciences, Family and Preventive Medicine
Chicago Medical School

Gordon, William
Assistant Professor
Interprofessional Healthcare Studies, Pre-Professional Health Sciences, Biomedical Sciences
College of Health Professions
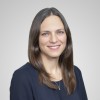
Graham, Hannah
Assistant Education Director
Clinical Sciences, Family and Preventive Medicine
Chicago Medical School
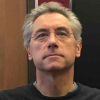
Gross, Adrian
Associate Professor
Foundational Sciences and Humanities, Biochemistry and Molecular Biology
Chicago Medical School, School of Graduate and Postdoctoral Studies

Grumet, Mark
Director of the Center for Clinical Anatomy, Associate Professor
Podiatric Basic Biomedical Sciences, Basic Biomedical Sciences
Dr. William M. Scholl College of Podiatric Medicine
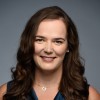
Haag, Sarah
Assistant Professor IPHS
Interprofessional Healthcare Studies, Physical Therapy
College of Health Professions

Hagopian, Kavork
Director of Clinical Education, Assistant Professor
Physical Therapy
College of Health Professions
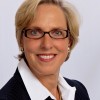
Hammerberg, Lucy
Assistant Dean for Career and Specialty Advising, Assistant Professor, Discipline Chair of Emergency Medicine
Student Affairs, Clinical Sciences, Medicine
Chicago Medical School
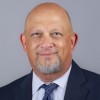
Hanes, Scott
Associate Dean of Clinical Affairs, Associate Professor
Pharmacy Practice
College of Pharmacy
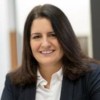
Hanson, Marilyn
Associate Vice President for Interprofessional Operations, Assistant Professor
Interprofessional Healthcare Studies
College of Health Professions

Harris, Edwin
Instructor
Podiatric Medicine & Surgery
Dr. William M. Scholl College of Podiatric Medicine

Harrison, David
Vice Chair of Pharmaceutical Sciences, Director of Academic Success, Professor
Pharmaceutical Sciences, Biochemistry and Molecular Biology, Foundational Sciences and Humanities
College of Pharmacy, School of Graduate and Postdoctoral Studies, Chicago Medical School
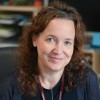
Hastings, Michelle
Professor & Director, Center for Genetic Diseases
Foundational Sciences and Humanities, Cell Biology and Anatomy
Chicago Medical School, School of Graduate and Postdoctoral Studies
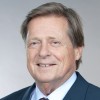
Hawkins, Richard
Professor Emeritus
Foundational Sciences and Humanities, Physiology and Biophysics
Chicago Medical School, School of Graduate and Postdoctoral Studies
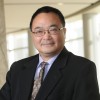
He, Johnny
Professor
Foundational Sciences and Humanities, Microbiology and Immunology
Chicago Medical School, School of Graduate and Postdoctoral Studies
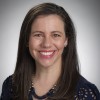
Henschen, Elizabeth
Assistant Education Director and Clinical Instructor
Clinical Sciences, Pediatrics
Chicago Medical School
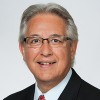
Herbig, Ronald
Assistant Professor, Lead Online Program Learning Experience Designer
Interprofessional Healthcare Studies, Nutrition
College of Health Professions
Hill, Evan
Research Assistant Professor
Biomedical Research
School of Graduate and Postdoctoral Studies
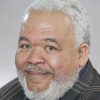
Horsley, Neil
Assistant Professor
Podiatric Medicine & Surgery
Dr. William M. Scholl College of Podiatric Medicine
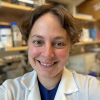
Howe, Erin
Assistant Professor
Biochemistry and Molecular Biology, Foundational Sciences and Humanities
Chicago Medical School, School of Graduate and Postdoctoral Studies

Hughes, Francis
Director of Pharmacy Skills Education, Associate Professor
Pharmacy Practice
College of Pharmacy
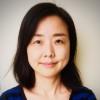
Hwang, EunJung
Assistant Professor
Foundational Sciences and Humanities, Cell Biology and Anatomy
Chicago Medical School, School of Graduate and Postdoctoral Studies

Intine, Robert
Associate Vice President for Academic and Faculty Affairs, Associate Dean of Research Education Programs & Associate Professor
Basic Biomedical Sciences
School of Graduate and Postdoctoral Studies

Jampala, V. Chowdary
Professor
Clinical Sciences, Psychiatry & Behavioral Science
Chicago Medical School

Jarrett, Beth
Associate Professor
Podiatric Medicine & Surgery
Dr. William M. Scholl College of Podiatric Medicine

Jayasanker, M.R.
Clinical Assistant Professor
Foundational Sciences and Humanities, Cell Biology and Anatomy
Chicago Medical School
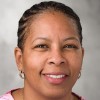
Jones, Monique
Associate Professor & Interim Assistant Dean for Career and Specialty Advising
Clinical Sciences, Obstetrics and Gynecology
Chicago Medical School
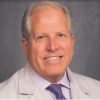
Jones, Paul
Clinical Associate Professor, Learning Community Mentor
Clinical Sciences, Surgery
Chicago Medical School
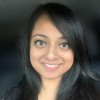
Joseph, Jincy
Assistant Professor & Learning Community Mentor
Family and Preventive Medicine, Clinical Sciences
Chicago Medical School
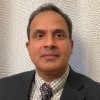
Joshi, Tilak
Assistant Professor
Podiatric Medicine & Surgery
Dr. William M. Scholl College of Podiatric Medicine

Juliano, Sheryl
Instructor & Assistant Dean for Curriculum and Instruction
Student Affairs, Foundational Sciences and Humanities, Humanities & Health Care
Chicago Medical School

Kam, Kaiwen
Associate Professor
Foundational Sciences and Humanities, Cell Biology and Anatomy
Chicago Medical School, School of Graduate and Postdoctoral Studies
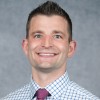
Kane, Sean
Senior Associate Dean, Associate Dean for Assessment, Professor
Pharmacy Practice
College of Pharmacy
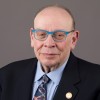
Kaplan, Ronald
Professor Emeritus
Foundational Sciences and Humanities, Biochemistry and Molecular Biology
School of Graduate and Postdoctoral Studies, Chicago Medical School

Kaplan, Bruce
Clinical Professor; Discipline Chair of Ophthalmology
Clinical Sciences, Surgery, Ophthalmology
Chicago Medical School
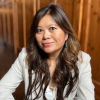
Kasalajtis, Lisa
Lecturer & Learning Community Mentor
Essentials of Clinical Reasoning, Clinical Sciences, Medicine
Chicago Medical School
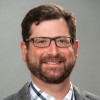
Katz, Ariel
Associate Professor and Education Director, Clinical Skills & Clinical Foundations of Medicine
Clinical Sciences, Medicine
Chicago Medical School
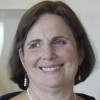
Katz, Rea
Adjunct Associate Professor, Volunteer Faculty
Physician Assistant
College of Health Professions
Kelly, Noreen
Clinical Assistant Professor
Foundational Sciences and Humanities, Cell Biology and Anatomy
Chicago Medical School
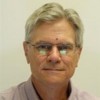
Kelly, Leo
Associate Professor & Director of Clinical Education
Clinical Sciences, Medicine
Chicago Medical School
Kessler, Elizabeth
Associate Professor of Psychiatry
Clinical Sciences, Psychiatry & Behavioral Science, Neurology
Chicago Medical School
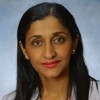
Khanna, Rakhi
Clinical Assistant Professor & Learning Community Mentor
Medicine, Clinical Sciences
Chicago Medical School
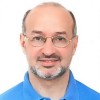
Khayr, Walid
Professor & Infectious Disease Fellowship Director
Clinical Sciences, Medicine, Physician Assistant
Chicago Medical School, College of Health Professions
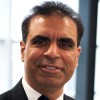
Khosla, Sandeep
Professor; Cardiovascular Disease Fellowship Director; Interventional Cardiology Fellowship Director
Clinical Sciences, Medicine
Chicago Medical School

Kiken, Stuart
Associate Professor, Discipline Chair, Education Director (Internal Medicine)
Clinical Sciences, Medicine
Chicago Medical School
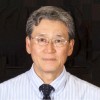
Kim, Donghee
Professor and Discipline Chair
Foundational Sciences and Humanities, Physiology and Biophysics
Chicago Medical School, School of Graduate and Postdoctoral Studies

Kim, Hongkyun
Associate Professor
Foundational Sciences and Humanities, Cell Biology and Anatomy
Chicago Medical School, School of Graduate and Postdoctoral Studies

Kim, Jean
Associate Professor, ECR Preceptor
Clinical Sciences, Pediatrics
Chicago Medical School

Klein, Erin
Clinical Instructor
Podiatric Medicine & Surgery
Dr. William M. Scholl College of Podiatric Medicine
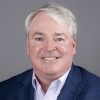
Knott, Patrick
Professor, Physician Assistant Department
Physician Assistant
College of Health Professions
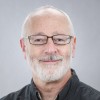
Kosson, David
Professor Emeritus
Psychology, Foundational Sciences and Humanities, Humanities & Health Care
School of Graduate and Postdoctoral Studies, Chicago Medical School, College of Health Professions
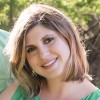
Kotlyar, Biana
Education Director, Discipline Chair, Associate Professor
Psychiatry & Behavioral Science
Chicago Medical School

Kwak-Kim, Joanne
Professor; Reproductive Immunology Specialist; Director of Reproductive Medicine and Immunology
Clinical Sciences, Obstetrics and Gynecology, Foundational Sciences and Humanities, Microbiology and Immunology
Chicago Medical School
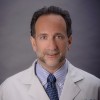
Lanoff, Martin
Associate Professor
Physical Med & Rehabilitation, Clinical Sciences
Chicago Medical School
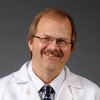
Latva, Darrell
Associate Professor
Podiatric Medicine & Surgery
Dr. William M. Scholl College of Podiatric Medicine
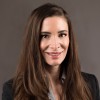
Lewis, Jessica
Assistant Dean for Student Affairs, Assistant Professor
Foundational Sciences and Humanities
Chicago Medical School
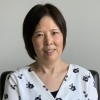
Liu, Ying
Research Professor
Foundational Sciences and Humanities, Microbiology and Immunology
Chicago Medical School
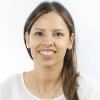
LoDuca, Ana
Associate Professor of Surgery
Clinical Sciences, Surgery, Ophthalmology
Chicago Medical School

Loomis, Patricia
Research Assistant Professor, Director Confocal Microscopy Lab
Biomedical Research, Foundational Sciences and Humanities, Cellular and Molecular Pharmacology
School of Graduate and Postdoctoral Studies, Chicago Medical School
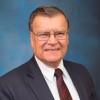
Lorenc, Z. Ted
Associate Professor & Clerkship Site Manager
Clinical Sciences, Medicine
Chicago Medical School

Lu, Min
Associate Professor
Foundational Sciences and Humanities, Biochemistry and Molecular Biology
Chicago Medical School, School of Graduate and Postdoctoral Studies
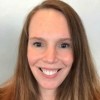
Lund, Amanda
Assistant Professor, Assistant Education Director (Internal Medicine), Co-Director for Palliative Care
Clinical Sciences, Medicine
Chicago Medical School
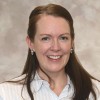
Lyden, Abbie
Associate Professor, Lead Interprofessional Faculty
Pharmacy Practice
College of Pharmacy

Mahr, Kristin
Assistant Professor, Director of Clinical Education, Physician Assistant Department, College of Health Professions
Physician Assistant
College of Health Professions
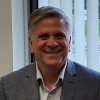
Maldonado, Frank
Professor & Executive Chair of Clinical Sciences
Clinical Sciences, Medicine
Chicago Medical School
Manion, Bruce
Professor Emeritus
Podiatric Basic Biomedical Sciences, Cell Biology and Anatomy, Basic Biomedical Sciences
Dr. William M. Scholl College of Podiatric Medicine, Chicago Medical School
Marasigan, Ligaya
Associate Professor
Clinical Sciences, Obstetrics and Gynecology
Chicago Medical School

Marr, Robert
Associate Professor & Assistant Dean for Research
Foundational Sciences and Humanities, Neuroscience
Chicago Medical School, School of Graduate and Postdoctoral Studies
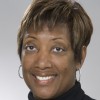
Mason, Karona
Vice Dean, Associate Professor
Podiatric Medicine & Surgery
Dr. William M. Scholl College of Podiatric Medicine
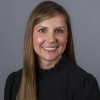
Mattes, Mary
Assistant Professor, Program Director & Chair
Pathologists' Assistant
College of Health Professions

McCormack, Charles
Professor
Foundational Sciences and Humanities, Physiology and Biophysics
Chicago Medical School
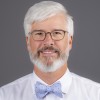
McShane, Franklin
Associate Professor, Director of Nursing Simulation
Nurse Anesthesia
College of Nursing

Michener, Lisa
Assistant Professor, Director of Clinical Development
Pharmacy Practice
College of Pharmacy
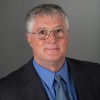
Moninger, Michael
Assistant Professor; Director of Academic Advising & Success
Foundational Sciences and Humanities, Humanities & Health Care, Student Affairs
Chicago Medical School
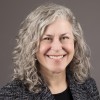
Morrison, Jeanette
Professor; Vice Dean for Medical Education, Executive Vice Dean for CMS
Clinical Sciences, Medicine, Medical Education
Chicago Medical School

Mosnaim, Aron
Professor
Foundational Sciences and Humanities, Cellular and Molecular Pharmacology, Clinical Sciences, Psychiatry & Behavioral Science
Chicago Medical School
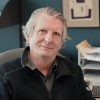
Mueller, David
Professor
Foundational Sciences and Humanities, Biochemistry and Molecular Biology
Chicago Medical School, School of Graduate and Postdoctoral Studies
Nair, Velayudhan
Emeritus Vice President for Research, Emeritus Dean School of Graduate and Postdoctoral Studies, Distinguished Professor of Pharmacology and Therapeutics
Cellular and Molecular Pharmacology
School of Graduate and Postdoctoral Studies, Chicago Medical School
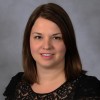
Ndiaye, Sandra
Co-Education Director for Pediatrics
Clinical Sciences, Pediatrics
Chicago Medical School
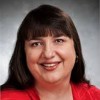
Nikolich, Sanja
Associate Professor & Education Director
Clinical Sciences, Surgery
Chicago Medical School
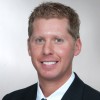
Nuciforo, Matthew
Chair and Program Director, Department of Physical Therapy, Associate Dean of Admissions and Enrollment, Associate Professor
Physical Therapy
College of Health Professions

O'Donnell, James
Associate Professor
Foundational Sciences and Humanities, Cellular and Molecular Pharmacology, Pharmaceutical Sciences
Chicago Medical School, College of Pharmacy

Oblinger, Monica
Professor Emeritus; Associate Vice President for Research Compliance
Foundational Sciences and Humanities, Cell Biology and Anatomy
Chicago Medical School, School of Graduate and Postdoctoral Studies
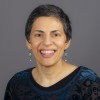
Oggoian, Rosanne
Assistant Professor; Clinical Skills Course Director & Lab Director
Essentials of Clinical Reasoning, Clinical Sciences, Pediatrics
Chicago Medical School

Oh, Kelly
Research Assistant Professor
Biomedical Research, Foundational Sciences and Humanities, Cell Biology and Anatomy
School of Graduate and Postdoctoral Studies, Chicago Medical School
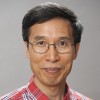
Oh, Kyoung
Associate Professor
Foundational Sciences and Humanities, Biochemistry and Molecular Biology
Chicago Medical School, School of Graduate and Postdoctoral Studies
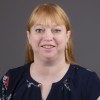
Olson, Maxine
Research Engineer, Lab Manager, Instructor
Physical Therapy
College of Health Professions
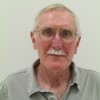
Oltmans, Gary
Professor Emeritus
Pharmaceutical Sciences, Cellular and Molecular Pharmacology
College of Pharmacy, Chicago Medical School, School of Graduate and Postdoctoral Studies
Orellana, Fernando
Assistant Professor for ECR & Affiliate Site Director
Clinical Sciences, Emergency Medicine
Chicago Medical School
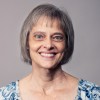
Pabst, Amy
Assistant Professor; Medical Director Healthcare Simulation
Clinical Sciences, Family and Preventive Medicine
Chicago Medical School
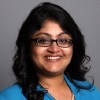
Pant, Nitika
Assistant Professor & Learning Community Mentor
Clinical Sciences, Psychiatry & Behavioral Science
Chicago Medical School
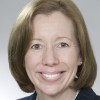
Parsley, Nancy
Provost, Associate Professor
Podiatric Medicine & Surgery
Dr. William M. Scholl College of Podiatric Medicine
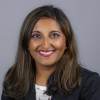
Patel, Pratibha
Associate Dean, Clerkship & Residency Placement, Assistant Professor
Podiatric Medicine & Surgery, Essentials of Clinical Reasoning, Clinical Sciences, Surgery
Dr. William M. Scholl College of Podiatric Medicine, Chicago Medical School
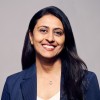
Patel-Mehta, Arpita
Discipline Chair, Education Director
Clinical Sciences, Family and Preventive Medicine
Chicago Medical School

Perlmuter, Lawrence
Professor Emeritus - Retired
Psychology, Clinical Sciences, Medicine
School of Graduate and Postdoctoral Studies, College of Health Professions, Chicago Medical School
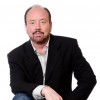
Peterson, Daniel
Professor; Director, Center for Stem Cell and Regenerative Medicine (CSCRM)
Foundational Sciences and Humanities, Neuroscience
Chicago Medical School, School of Graduate and Postdoctoral Studies
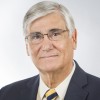
Peterson, Darryl
Professor Emeritus
Foundational Sciences and Humanities, Physiology and Biophysics, Clinical Sciences, Medicine
Chicago Medical School, School of Graduate and Postdoctoral Studies
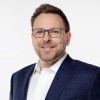
Phelps, Paul
Clinical Associate Professor
Clinical Sciences, Surgery, Ophthalmology
Chicago Medical School

Pilch, Liza
Assistant Professor & Learning Community Mentor
Clinical Sciences, Emergency Medicine
Chicago Medical School
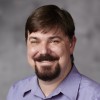
Polanski, Joshua
Associate Professor
Foundational Sciences and Humanities, Cell Biology and Anatomy
Chicago Medical School
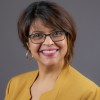
Pomarico, Yovanna
Assistant Professor
Biomedical Sciences, Pre-Professional Health Sciences
College of Health Professions

Potashkin, Judith
Professor and Discipline Chair
Foundational Sciences and Humanities, Cellular and Molecular Pharmacology
Chicago Medical School, School of Graduate and Postdoctoral Studies
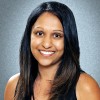
Prasad-Reddy, Lalita
Associate Professor, Discipline Chair of Humanities & Health Care
Cellular and Molecular Pharmacology, Foundational Sciences and Humanities, Humanities & Health Care
Chicago Medical School

Radke, Jason
Assistant Professor, Chair & Program Director
Physician Assistant
College of Health Professions

Raj, Garud
Clinical Assistant Professor
Foundational Sciences and Humanities, Cell Biology and Anatomy
Chicago Medical School
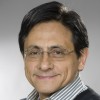
Rasgado-Flores, Hector
Professor
Foundational Sciences and Humanities, Physiology and Biophysics, Interprofessional Healthcare Studies
Chicago Medical School, School of Graduate and Postdoctoral Studies, College of Health Professions
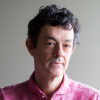
Re, Fabio
Professor
Foundational Sciences and Humanities, Microbiology and Immunology
School of Graduate and Postdoctoral Studies, Chicago Medical School

Reynolds, Joseph
Associate Professor; Director, Center for Cancer Cell Biology, Immunology and Infection; Discipline Chair, Microbiology and Immunology, Interim Vice Dean for Research; CMS
Foundational Sciences and Humanities, Microbiology and Immunology
Chicago Medical School, School of Graduate and Postdoctoral Studies

Rheault, Wendy
President, CEO, and Professor
Physical Therapy, Interprofessional Healthcare Studies
College of Health Professions
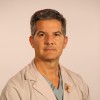
Rodriguez-Collazo, Edgardo
Assistant Professor, Director of Outreach Strategies
Podiatric Medicine & Surgery
Dr. William M. Scholl College of Podiatric Medicine
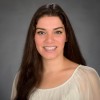
Rohani, Roxane
Assistant Professor
Cellular and Molecular Pharmacology, Foundational Sciences and Humanities
Chicago Medical School
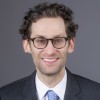
Rosenblatt, Noah
Associate Professor, Associate Dean of Research
Podiatric Medicine & Surgery
Dr. William M. Scholl College of Podiatric Medicine
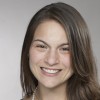
Rosenkranz, Jolee
Associate Dean for External Relations, Instructor
Pharmacy Practice
College of Pharmacy

Rosenkranz, Jeremy
Professor; Director, Brain Science Institute
Foundational Sciences and Humanities, Cellular and Molecular Pharmacology
Chicago Medical School, School of Graduate and Postdoctoral Studies

Sackin, Henry
Professor
Foundational Sciences and Humanities, Physiology and Biophysics
Chicago Medical School, School of Graduate and Postdoctoral Studies
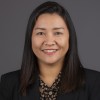
Saengsawang, Witchuda
Assistant Professor
Basic Biomedical Sciences
Dr. William M. Scholl College of Podiatric Medicine
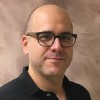
Sandalow, Nathan
Clerkship Director & Associate Professor
Clinical Sciences, Emergency Medicine
Chicago Medical School
Sarma, Bala
Associate Professor
Clinical Sciences, Psychiatry & Behavioral Science
Chicago Medical School
Sarras Jr., Michael
Professor Emeritus
Foundational Sciences and Humanities, Cell Biology and Anatomy
Chicago Medical School, School of Graduate and Postdoctoral Studies, College of Health Professions
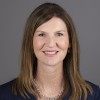
Scanlon, M. Susan
Assistant Professor & Learning Community Mentor
Clinical Sciences, Medicine
Chicago Medical School
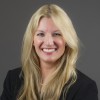
Schnack, Lauren
Assistant Professor
Podiatric Medicine & Surgery
Dr. William M. Scholl College of Podiatric Medicine
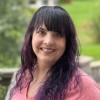
Schulte, Suzanne
Discipline Chair and Co-Education Director for Pediatrics
Clinical Sciences, Pediatrics
Chicago Medical School
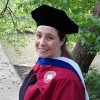
Schwertfeger, Julie
Clinical Assistant Professor, Lecturer
Clinical Sciences, Psychiatry & Behavioral Science, Interprofessional Healthcare Studies
Chicago Medical School, College of Health Professions
Seidenberg, Michael
Professor Emeritus - Retired
Psychology, Clinical Sciences, Psychiatry & Behavioral Science
College of Health Professions, Chicago Medical School, School of Graduate and Postdoctoral Studies

Senno, Ricardo
Assistant Professor
Clinical Sciences, Physical Med & Rehabilitation
Chicago Medical School
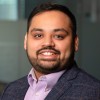
Shah, Deep
Assistant Professor
Podiatric Medicine & Surgery
Dr. William M. Scholl College of Podiatric Medicine
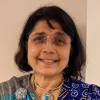
Shah, Pallavi
Clinical Associate Professor & Learning Community Mentor
Medicine, Clinical Sciences
Chicago Medical School

Sharma-Walia, Neelam
Associate Professor
Foundational Sciences and Humanities, Microbiology and Immunology
Chicago Medical School, School of Graduate and Postdoctoral Studies
Siegel, Lori
Medical Director Physician Assistant Program, Clinical Professor
Physician Assistant, Medicine
College of Health Professions, Chicago Medical School
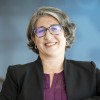
Simanek, Amanda
Director, Michael Reese Foundation Center for Health Equity Research; Associate Professor, Epidemiology
Foundational Sciences and Humanities, Humanities & Health Care
Chicago Medical School
Simon, Edwin
Associate Professor
Clinical Sciences, Psychiatry & Behavioral Science, Medicine
Chicago Medical School

Skladzien, Gregory
Assistant Professor
Physician Assistant, Foundational Sciences and Humanities, Cell Biology and Anatomy
College of Health Professions, Chicago Medical School
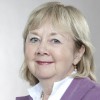
Snyder, Ann
Professor Emeritus
Foundational Sciences and Humanities, Cellular and Molecular Pharmacology, Clinical Sciences, Medicine
Chicago Medical School, School of Graduate and Postdoctoral Studies
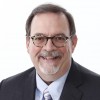
Sowers, Bruce
Assistant Professor
Interprofessional Healthcare Studies
College of Health Professions

Srivastava, Sneha
Associate Director of Pharmacy Skills Education, Associate Professor
Pharmacy Practice
College of Pharmacy
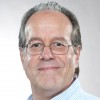
Steiner, Heinz
Professor
Foundational Sciences and Humanities, Cellular and Molecular Pharmacology
Chicago Medical School, School of Graduate and Postdoctoral Studies

Stevens, Karen
Associate Professor, Assistant Program Director
Physical Therapy
College of Health Professions

Stiles, William
Clinical Assistant Professor
Clinical Sciences, Surgery, Ophthalmology
Chicago Medical School
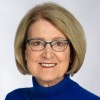
Stoecker, Judith
Associate Professor
Physical Therapy, Interprofessional Healthcare Studies
College of Health Professions
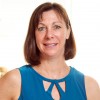
Stutzmann, Grace
Professor & Discipline Chair of Neuroscience; Director, Center for Neurodegenerative Disease and Therapeutics
Foundational Sciences and Humanities, Neuroscience
Chicago Medical School, School of Graduate and Postdoctoral Studies
Sukowski, Ernest
Associate Professor Emeritus
Physiology and Biophysics, Pre-Professional Health Sciences
Chicago Medical School, College of Health Professions
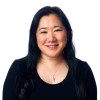
Suzue, Kimiko
Professor, Education Director and Discipline Chair, Pathology
Clinical Sciences, Pathology, Podiatric Basic Biomedical Sciences
Chicago Medical School, Dr. William M. Scholl College of Podiatric Medicine
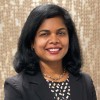
Swaminathan, Bharathi
Discipline Chair and Associate Professor, PM&R; EMG Fellowship Director; Site Director, PM&R Residency Program
Clinical Sciences, Physical Med & Rehabilitation
Chicago Medical School

Swift-Johnson, Amy
Associate Professor
Clinical Sciences, Family and Preventive Medicine, Essentials of Clinical Reasoning
Chicago Medical School
Symersky, Jindrich
Research Assistant Professor
Biomedical Research
School of Graduate and Postdoctoral Studies
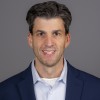
Talbot, Derek
Chair, Department of Basic Biomedical Sciences, Associate Professor
Basic Biomedical Sciences
Dr. William M. Scholl College of Podiatric Medicine
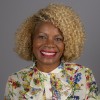
Taylor, Erica
Assistant Professor and Learning Community Mentor
Clinical Sciences, Obstetrics and Gynecology
Chicago Medical School
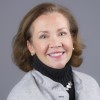
Thuente, Lori
Director, Scholl Family Foundation Empathy Lab
Interprofessional Healthcare Studies, Entry into Nursing Practice
College of Nursing, College of Health Professions
Tierney, Kristine
Assistant Professor, Coordinator of Clinical Education
Nurse Anesthesia
College of Nursing
Tokarczyk, Sheri
Lecturer
Physician Assistant
College of Health Professions
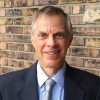
Tomchik, Kenneth
Lecturer, Learning Community Mentor
Clinical Sciences, Medicine
Chicago Medical School
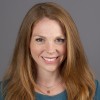
Toner, Lauren
Instructor, Director of Clinical Education
Physical Therapy
College of Health Professions
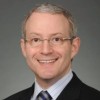
Trester, Richard
Associate Professor; Discipline Chair, Education Director
Clinical Sciences, Obstetrics and Gynecology
Chicago Medical School
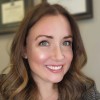
Turrentine, Natalie
Assistant Professor, Assistant Program Director for Student Success
Physical Therapy
College of Health Professions

Urban, Janice
Director, Center for Neurobiology of Stress Resilience and Psychiatric Disorders; Professor, Physiology and Biophysics
Foundational Sciences and Humanities, Physiology and Biophysics
Chicago Medical School, School of Graduate and Postdoctoral Studies
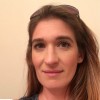
Vantrease, Jaime
Research Assistant Professor
Foundational Sciences and Humanities, Physiology and Biophysics
Chicago Medical School

Vedak, Chandra
Clinical Associate Professor
Clinical Sciences, Psychiatry & Behavioral Science
Chicago Medical School
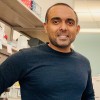
Vijay, Rahul
Assistant Professor
Foundational Sciences and Humanities, Microbiology and Immunology
Chicago Medical School, School of Graduate and Postdoctoral Studies
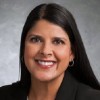
Wagle, Smriti (Simi)
Assistant Education Director & Assistant Professor
Clinical Sciences, Neurology
Chicago Medical School
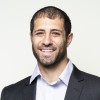
Wahhab, John
Emergency Medicine Sub-Internship Director & Assistant Professor
Clinical Sciences, Emergency Medicine
Chicago Medical School

Walters, Eric
Professor
Pharmaceutical Sciences, Foundational Sciences and Humanities, Biochemistry and Molecular Biology
Chicago Medical School, School of Graduate and Postdoctoral Studies, College of Pharmacy
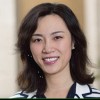
Wang, Ashley
Assistant Professor
Clinical Sciences, Medicine
Chicago Medical School
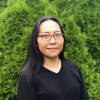
Wang, Ning
Associate Professor
Foundational Sciences and Humanities, Microbiology and Immunology
Chicago Medical School, School of Graduate and Postdoctoral Studies
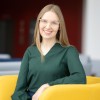
Waszczuk, Monika
Associate Professor
Psychology, Foundational Sciences and Humanities, Neuroscience
College of Health Professions, Chicago Medical School

Weiand, Steven
Associate Dean for Student Affairs, Chair & Assistant Professor
Biomedical Sciences, Interprofessional Healthcare Studies, Pre-Professional Health Sciences, Physical Therapy
College of Health Professions
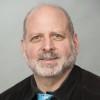
Weisenberg, Elliot
Associate Professor and Medical Director
Pathologists' Assistant, Clinical Sciences, Pathology
College of Health Professions, Chicago Medical School
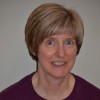
Wellington, Christine
Assistant Professor
Humanities & Health Care, Foundational Sciences and Humanities, Nutrition
Chicago Medical School, College of Health Professions

Winnike, Janeen
Associate Dean for Student Affairs, Assistant Professor
Pharmacy Practice
College of Pharmacy

Woodard, Qeena
Associate Dean of Clinical Experiences, Associate Professor
Podiatric Medicine & Surgery
Dr. William M. Scholl College of Podiatric Medicine
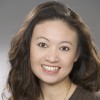
Wu, Stephanie
Dean, Professor
Podiatric Medicine & Surgery, Center for Stem Cell and Regenerative Medicine
Dr. William M. Scholl College of Podiatric Medicine, School of Graduate and Postdoctoral Studies

Yalla, Sai
Assistant Professor
Podiatric Medicine & Surgery
Dr. William M. Scholl College of Podiatric Medicine
Yang, Xinli
Research Assistant Professor
Biomedical Research
School of Graduate and Postdoctoral Studies

Yau, Sydney
Interim Chair, Associate Dean of Educational Affairs, Associate Professor
Podiatric Medicine & Surgery
Dr. William M. Scholl College of Podiatric Medicine

Zdon, Michael
Professor, Discipline Chair of Surgery, Senior Associate Dean for GME and CME, Designated Institutional Official (DIO), Vice Chair of Clinical Sciences
Clinical Sciences, Surgery, Medicine
Chicago Medical School

Zun, Leslie
Professor; Director of Clinical Faculty Development
Clinical Sciences, Emergency Medicine, Psychiatry & Behavioral Science
Chicago Medical School